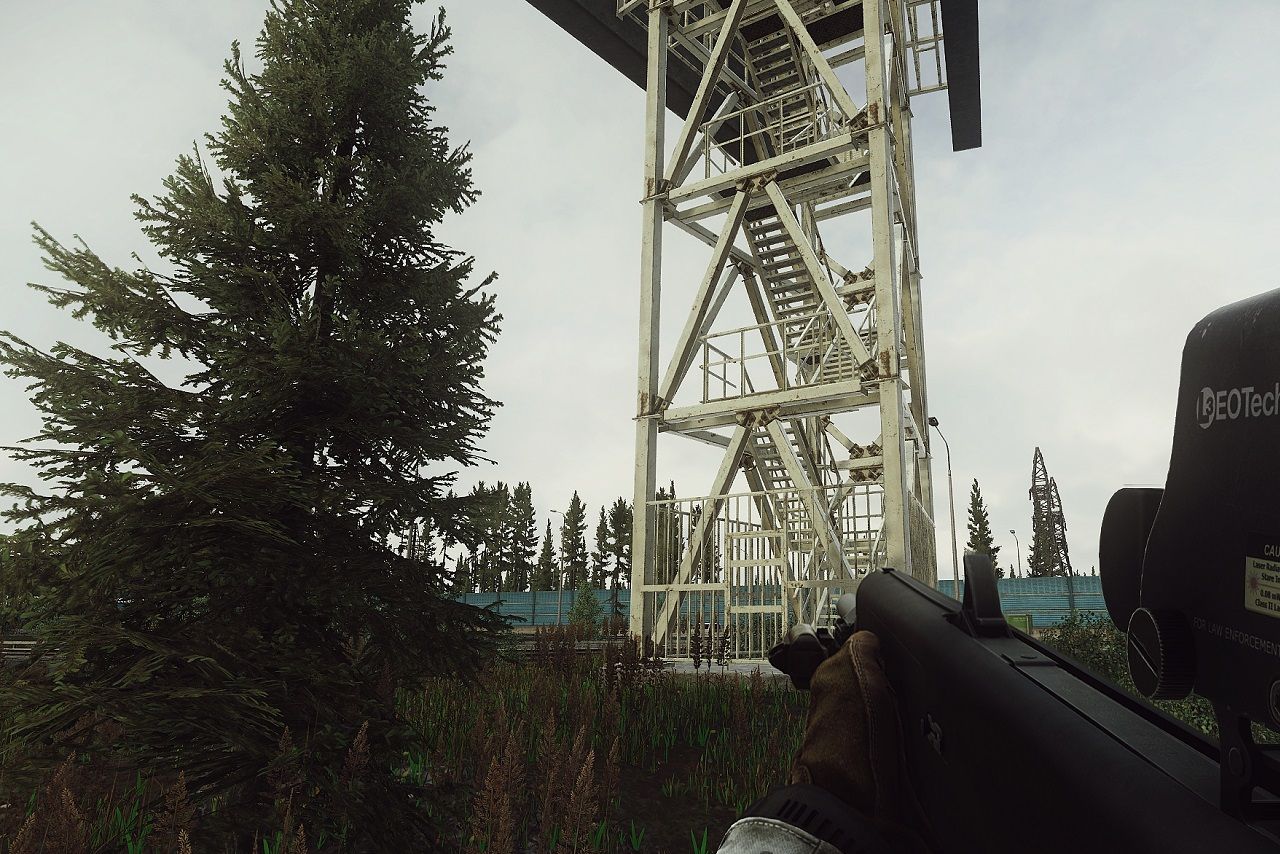
Anti-Aliasing: An In-Depth Guide to Better Graphics in Games
|
In this article, we’ll break down the nature of aliasing, analyze the basic principles of anti-aliasing, and help you make informed choices when selecting anti-aliasing methods for in-game graphics settings or game development.
Table of Contents
- Introduction
- What is Aliasing
- How Anti-Aliasing Works
- Types of Anti-Aliasing Methods
- Quality and Efficiency of Anti-Aliasing Methods
- Development Trends and Prospects
- Anti-Aliasing Settings for Gamers
- List of Common Misconceptions
- TL;DR
Introduction
Anti-aliasing is a set of methods and techniques used in 2D and 3D computer graphics and digital image processing to eliminate or mitigate visual distortions that occur when converting continuous signals into digital form. Simply put, when we try to display diagonal lines, curves, or small details on a screen made up of square pixels, noticeable stair-like lines (jaggies) appear at the boundaries of objects. This effect is called aliasing.
This became relevant simultaneously with the advent of the first electronic displays and video games, when the screen resolution was low and the possibilities for rendering details were minimal. However, even in the modern era of high-resolution monitors (Full HD, 4K) and powerful graphics cards, people continue to encounter distortions at the edges of objects. These distortions often make it difficult to perceive visual content the way its authors intended, and they can create an impression of a raw or low-quality picture.
Anti-aliasing is designed to make the image smoother and more natural, reducing jagged edges, and improving the overall visual quality. At the same time, there are different methods and approaches to anti-aliasing, each of which has its own advantages and disadvantages.
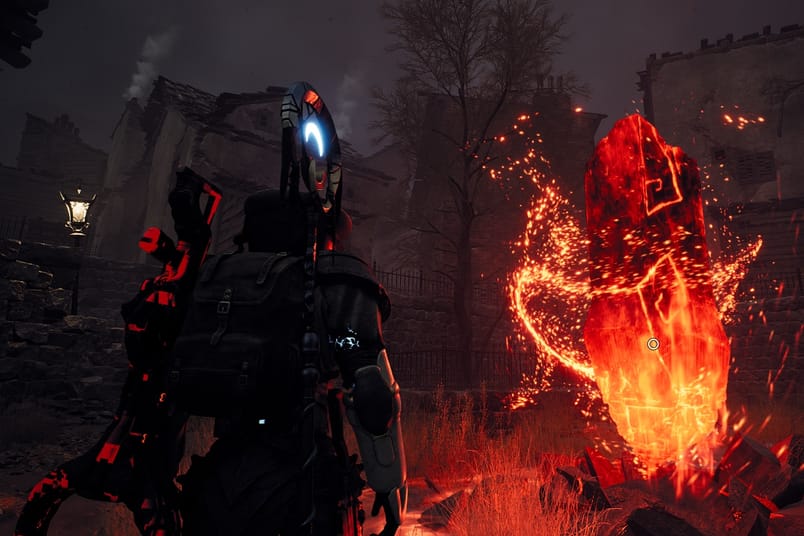
What is Aliasing
In order to better understand “what is anti-aliasing?”, we first need to break down aliasing (also known as jagging). It is manifested when an image consisting of discrete pixels attempts to display a continuous line (or the border of an object). Since pixels have a fixed shape (usually square) and a predefined position in the raster, any diagonal or curved structure is ruined when placed on the grid. In other words, this misalignment causes edges to look jagged, especially when the object’s color sharply contrasts with the background.
Imagine a straight line running at an angle to the horizontal. On paper, where we have infinite resolution (since paper isn’t made of pixels), the line will look smooth. But if you overlay a grid of pixels on it, then at each step of the line you need to choose which cells will be filled in and which will not. And naturally, some cells will be filled in incompletely with the line. Pixels can only use binary logic—either filled (color) or not filled (background)—which can lead to visual artifacts.
The problem is based on the continuous signal sampling process. According to the Nyquist—Shannon theorem, to correctly digitize a signal without losing information, the sampling rate must be at least twice as high as the maximum frequency present in the signal. In the case of graphics, the signal is the visual details and contours, and the frequency is the rate of color changes across the image. In simple terms, when the resolution isn’t high enough (or the tilt angle, contrast, or sharp transitions are too large), some small details get distorted, leading to jagging.
There are different areas, where aliasing can occur:
- 2D graphics—texts with distorted fonts and outlines, tilted icons, logos with jagged borders.
- 3D graphics—objects and models projected onto a two-dimensional screen (where polygons, especially those with angled edges, become sources of jaggies).
- Video and animation—dynamic aliasing when the camera or objects move, when the jagging of contours becomes especially noticeable in motion.
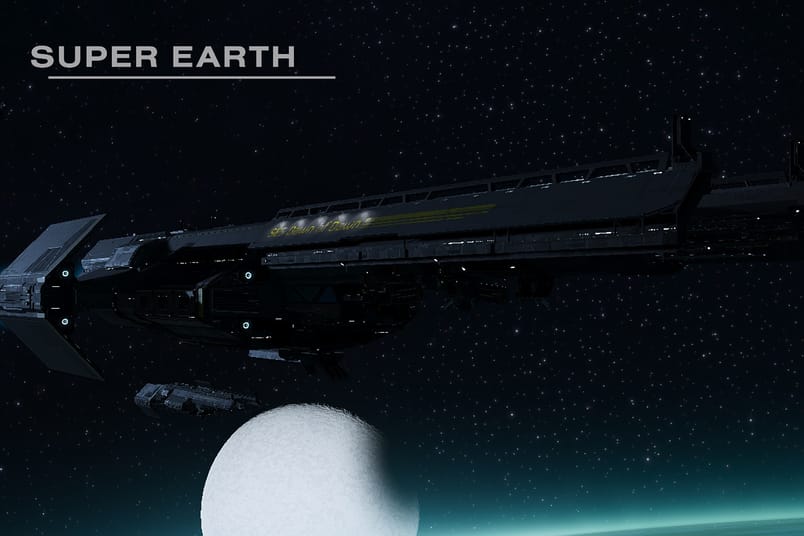
How Anti-Aliasing Works
The purpose of anti-aliasing is to fill in the missing information about subpixel (smaller than one pixel) details and borders so that the final image looks smoother.
The easiest way to eliminate the sharp transition between neighboring pixels that belong to different color areas is to use intermediate shades. If the true border of the line runs in the middle of a pixel, it is logical to set this pixel to a color mixed from the color of the object and the background in a certain proportion. This way, we will visually smooth out the sharp transition line.
One of the fundamental techniques of anti-aliasing is supersampling (or SSAA). It assumes that the image is being rendered (or processed) at higher resolution than required at the output. Then the resulting image is compressed to the target size, and during compression, filtering is applied, which allows you to get intermediate colors.
Any anti-aliasing method increases the computational load to a certain degree. Supersampling is especially heavy for your PC because it requires rendering an additional sizable number of pixels. Other methods (that analyse and change pre-rendered frames) are usually less resource-intensive, but the final quality may be slightly lower.
For many years, GPUs have built-in mechanisms that simplify the implementation of various forms (which we are about to analyze) of MSAA. This is a hardware optimization. There are also software solutions (FXAA, SMAA), which developers can integrate as post-processing shaders.
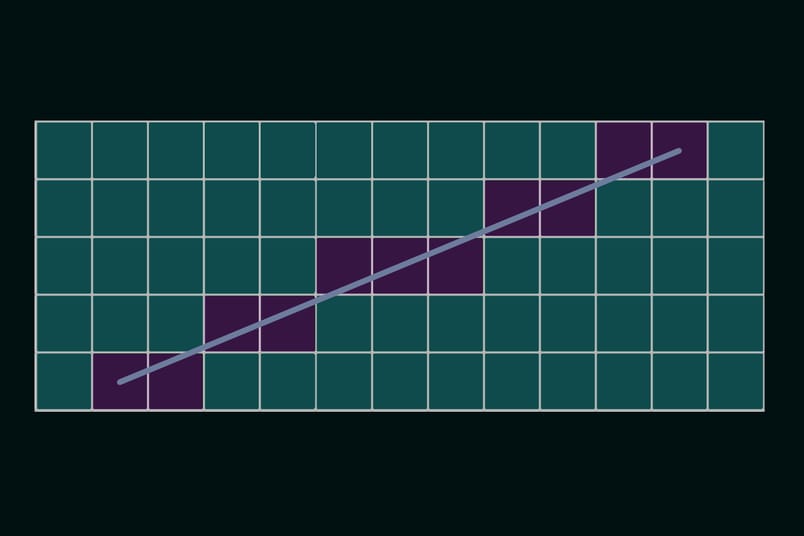
Types of Anti-Aliasing Methods
In practice, many different techniques are used. Let’s look at the main methods, from the classic heavyweight ones to the fast ones (focused on post-processing and using artificial intelligence to speed up the process and reduce performance costs).
Supersampling anti-aliasing (SSAA)
We render the scene at a resolution a few times higher than the target along each axis (resulting in 4-16 times more pixels), then downscale by averaging several neighboring pixels to form each one output pixel. This produces the best quality, as the entire frame is smoothed and all details are taken into account, but the load on CPU and GPU are proportionally higher.
Multisample anti-aliasing (MSAA)
The main objective of this method is to reduce the computational cost of SSAA. During rendering, only a few samples at the edges of polygons are taken into account (where aliasing is most noticeable). This means that the geometric information about the object’s boundary is captured with high accuracy, but the color data inside each pixel is not processed multiple times. The resulting quality at the polygon boundaries is high, and the resource consumption is lower in comparison to SSAA. But, it can introduce problems with texture transparency and some other graphical artifacts because certain effects are not properly handled.
Enhanced quality anti-aliasing (EQAA) and coverage sampled anti-aliasing (CSAA)
Iterations of MSAA from AMD and NVIDIA respectively. They increase the number of coverage samples without proportionally increasing the number of color samples. This way, you can define a more precise border shape without rendering all the pixels repeatedly. These methods are better than classic MSAA (a slight boost in sampling efficiency), but they have the same downsides.
Fast approximate anti-aliasing (FXAA)
Rendering speed increased by making anti-aliasing a part of post-processing. The algorithm analyzes the already generated frame (in the buffer) and tries to detect jagging based on contrast. After detecting, it applies a blurring effect to visually remove the harsh transitions. It works very fast, puts almost no load on the GPU, and is easily integrated into most engines. But it can blur subtle details (fonts or textures with small elements) and doesn’t take into account the depth of the scene.
Enhanced subpixel morphological anti-aliasing (SMAA)
Improvement of FXAA’s ideas—more accurate edge detection and usage of improved detection patterns. Produces less blurring and better smoothing of subpixel details, but requires more computational power than FXAA. Also, still not ideal for large brightness contrasts or complex lighting.
Temporal anti-aliasing (TAA)
To reduce graphical noise in motion, this method compares the current frame with the previous ones using data from a frame history buffer. In fact, each pixel is smoothed based on data from previous frames, which helps eliminate random jagging and noise. Downside of this algorithm is ghosting, as it may include outdated information or misinterpret movement. Also, sometimes there is an additional blurriness when the camera moves too quickly.
Methods based on neural networks (DLAA, DLSS, FSR, XeSS)
Most of these methods have already been discussed in the other article. The bottom line is to use machine learning algorithms to reconstruct a higher resolution image from a lower one, and combine it with smoothing. Deep learning anti-aliasing (DLAA) focuses purely on edge smoothing rather than upscaling, meaning it operates at native resolution. This results in superior image quality but comes with a substantial performance cost. Also, different methods may require specific hardware, drivers, or software support.
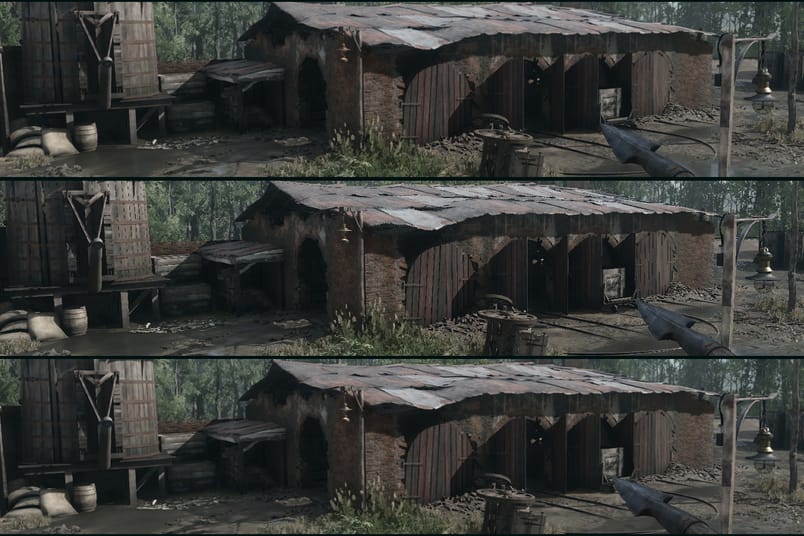
Quality and Efficiency of Anti-Aliasing Methods
Each smoothing approach has an area in which it shows the best results, as well as limitations that may be critical in some cases.
A comparative look at quality:
- SSAA is traditionally considered the best in terms of quality, as it misses the least details.
- MSAA is a solid compromise for desktop PCs, it provides good smoothing of the main geometric edges. However, it doesn’t always handle transparency and shaders well.
- FXAA and SMAA are ideal for projects where speed is important, where jagging needs to be eliminated quickly. The disadvantage is the possible blurring of details.
- TAA is especially effective if the goal is to eliminate aliasing during movement. In static scenes, TAA may lose compared to sharper solutions, but it looks better in dynamics.
- DLAA simply provides superior quality.
And efficiency:
- SSAA significantly increases the number of pixels for calculation, which can consume most of the resources even on powerful systems.
- MSAA requires more memory for samples and additional GPU power, but it is much more efficient compared to the previous method (rendering the entire frame in high resolution).
- Post-processing algorithms (FXAA, SMAA) operate on an already rendered frame, considerably reducing performance costs.
- TAA uses data from previous frames, requiring careful buffer management, but this is usually not too demanding for modern GPUs.
- DLAA imposes a higher performance cost due to its increased computational demand.
On monitors with high pixel density (4K and higher) aliasing becomes less noticeable, but it does not disappear completely. However, on large displays, jagging will still be visible because diagonal lines remain aligned to the pixel grid.
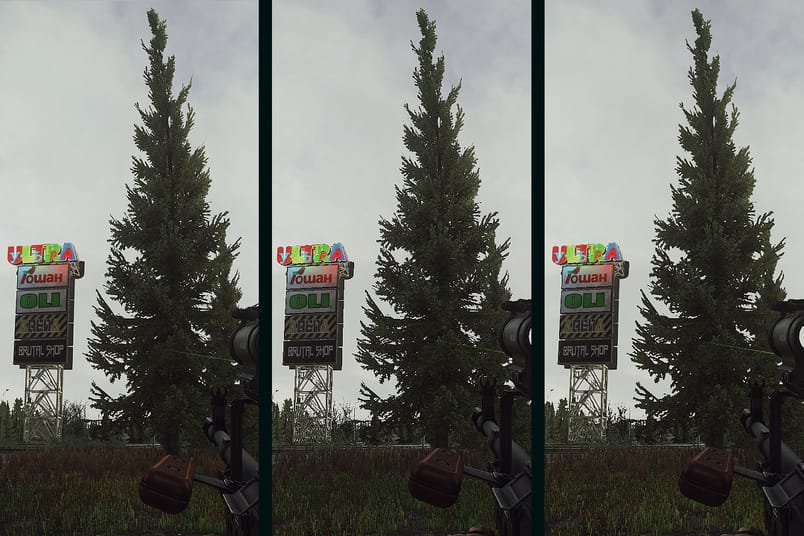
Development Trends and Prospects
The fields of computer graphics and game development are constantly evolving: new techniques, improved algorithms, and hardware capabilities are emerging.
Algorithms with machine learning. NVIDIA’s DLSS has already pushed the idea of smart upscaling far ahead. Using neural networks makes it possible to fill in details that are not present in the original resolution, at the same time reducing performance costs. FSR and XeSS are alternatives that run on a wider range of hardware, which makes it accessible to more users. Further evolution of more personalized iterations optimized for specific engines or games is likely, with models tuned to certain artistic or performance needs.
Hybrid solutions. Game engines are starting to combine TAA and machine learning algorithms to get the best of both worlds: dynamic smoothing and clarity enhancement through upscaling.
Hardware acceleration and GPU specialization. Modern graphics cards (NVIDIA RTX or AMD RDNA series) have specialized blocks for computing related to artificial intelligence. This means that GPU-based deep learning algorithms are becoming a reality for the mass user.
The future of ultra-high resolutions. Resolutions like 4K and higher theoretically reduce the visibility of aliasing, but require tremendous power for native rendering. Therefore, it is most likely that hybrid solutions (upscaling + anti-aliasing) will be the most popular choice, which allows achieving a visual 4K or even 8K without full rendering in these resolutions.
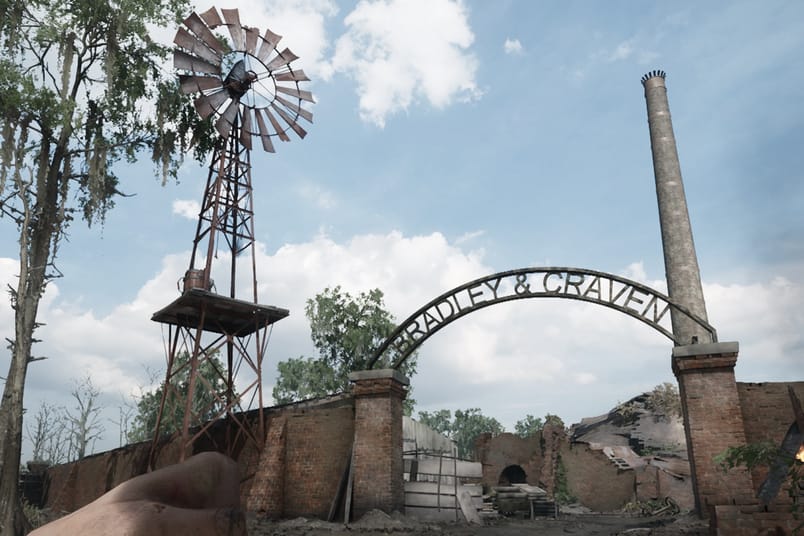
Anti-aliasing Settings for Gamers
Achieving a balanced combination of image quality and performance in modern games often requires attention to several factors, so consider the following.
System performance:
- High-end GPUs. For stable performance in demanding AAA titles at high resolution, it is advisable to use TAA or MSAA (4x–8x). Modern neural network techniques are also an option when hardware is sufficiently powerful.
- Lower-end or integrated graphics. In systems with limited resources, FXAA is often preferred for maintaining acceptable performance levels (at the cost of some sharpness).
Type of game:
- Fast-paced or open world games. TAA is often recommended here, as it smooths out jaggies in motion and prevents flickering edges. If the game supports upscaling with DLSS/FSR, this can further improve smoothness and clarity.
- Static scenes. In rare cases (for example, if you use the game as a benchmark for beautiful screenshots), you can try SSAA, as it gives the sharpest results. But in normal gameplay, it is too heavy and rarely relevant.
- Mobile or VR/AR games. Lightweight post-process methods (FXAA, etc) or adaptive anti-aliasing tend to be the most efficient choices for these.
Screen resolution and size:
- High DPI displays. When pixel density is elevated, visual artifacts are less pronounced, which may allow a lower anti-aliasing setting without compromising clarity.
- Lower resolutions (720p or below). Even simple post-processing filters (FXAA, SMAA) can significantly reduce harsh edges and improve the overall image.
Rendering features in specific games:
- Transparency. Scenes containing abundant foliage, glass, or smoke require more specialized anti-aliasing methods for better visibility, so it’s better to experiment between MSAA and FXAA/SMAA.
- Bright, high-contrast environments. HDR or other intense lighting setups may amplify blurring and jagging. Solutions with adjustable sharpening help maintain clarity (SMAA, TAA, etc).
Machine learning-based approaches (DLSS, FSR) offer an efficient alternative to traditional anti-aliasing by providing higher sharpness with lower performance costs. As these technologies continue to evolve, they may require specific driver updates or compatible hardware to achieve optimal results.
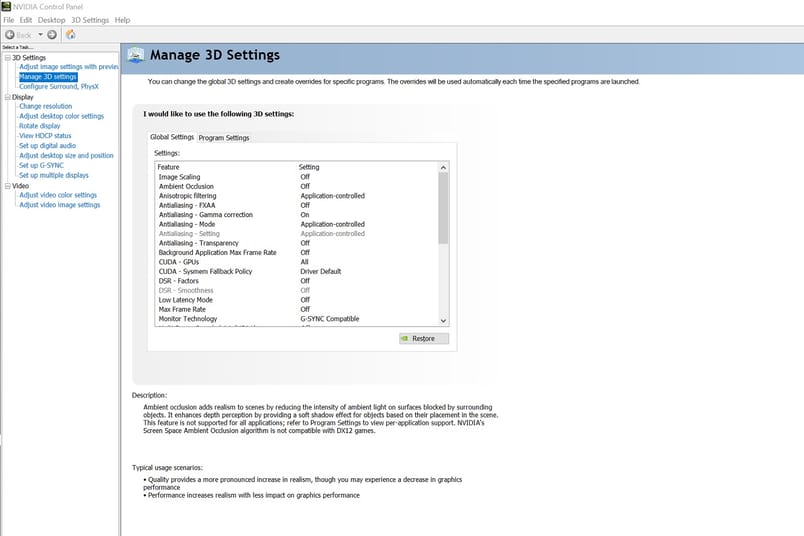
List of Common Misconceptions
Often, even in the professional environment, there are some misconceptions about anti-aliasing. Below are the most popular ones.
The higher the resolution, the less anti-aliasing is needed
Yes, it’s somewhat harder to notice the jagging at high resolutions. However, if contrasting lines and objects are positioned at an acute angle, the effect will still be visible, especially when moving. And this also depends on the actual size of a display.
FXAA gives the same quality as MSAA or TAA, but faster
Yes, FXAA is indeed faster, but its quality is usually inferior to geometrically oriented (MSAA) and temporal (TAA) methods. FXAA works with an already rendered frame and often blurs texture details.
Temporal methods are always better than static anti-aliasing
Yes, if you look at dynamic scenes, temporary smoothing often gives good results and reduces noise. But TAA can produce undesirable artifacts (blurring and ghosting).
DLSS is only usable for upscaling, not smoothing the image
No, DLSS and similar solutions from AMD and Intel go beyond simple upscaling by utilizing advanced algorithms to reconstruct the image. As a result, these methods combine resolution enhancement with anti-aliasing.
TL;DR
Anti-aliasing is a fundamental technology that stands at the intersection of mathematics, computer science, and optics. Its goal is to make computer graphics more visually appealing and to smooth out the jaggedness and other distortions that can occur. Despite the appearance of displays with 4K+ resolutions, the problem of aliasing is still relevant, because some angles and details will always produce a jagged effect when sampled.
Aliasing was examined from theory to practical issues like jagged edges. Various anti-aliasing methods were covered: SSAA and MSAA, hybrid solutions, post-processing techniques (FXAA, SMAA), and modern approaches like TAA and DLAA. Each of these methods has its own scope of use. Some deliver near-perfect quality at the cost of high resource consumption, while others are much faster with minimal GPU load, but at the expense of blurring or other artifacts.
The choice of the anti-aliasing method depends on many factors: the hardware capabilities of the target platform, the genre of the game or the type of graphical application, the size and resolution of the screen, the personal preferences of the developer or the user. There is no universal solution that would be the best in all aspects.
Current trends point to the active development of methods using machine learning and specialized hardware blocks in video cards. They are already achieving impressive results with relative resource savings, and, given the continuous improvement of neural network models, we can expect further improvements in the quality of image reconstruction and contour smoothing.
However, experience shows that older methods are not going away either. Depending on the task and conditions (especially in the mobile and web segment) they may remain the most convenient choice. Developers often combine several approaches: they use MSAA for key objects, complement TAA to smooth out dynamics, and post-processing methods as an additional touch to remove small jaggies at the final stage of rendering.
Ultimately, anti-aliasing should be seen as a comprehensive set of tools that shape both the perception and the quality of graphics. A competent understanding of how it works, as well as specifics of implementation, will enhance visual quality, making it more refined and aesthetically pleasing. The constant development of this field ensures that in the future we will be able to get an increasingly realistic picture. And maybe someday, resolutions and filters will become so advanced that aliasing will no longer be an issue for mainstream applications.